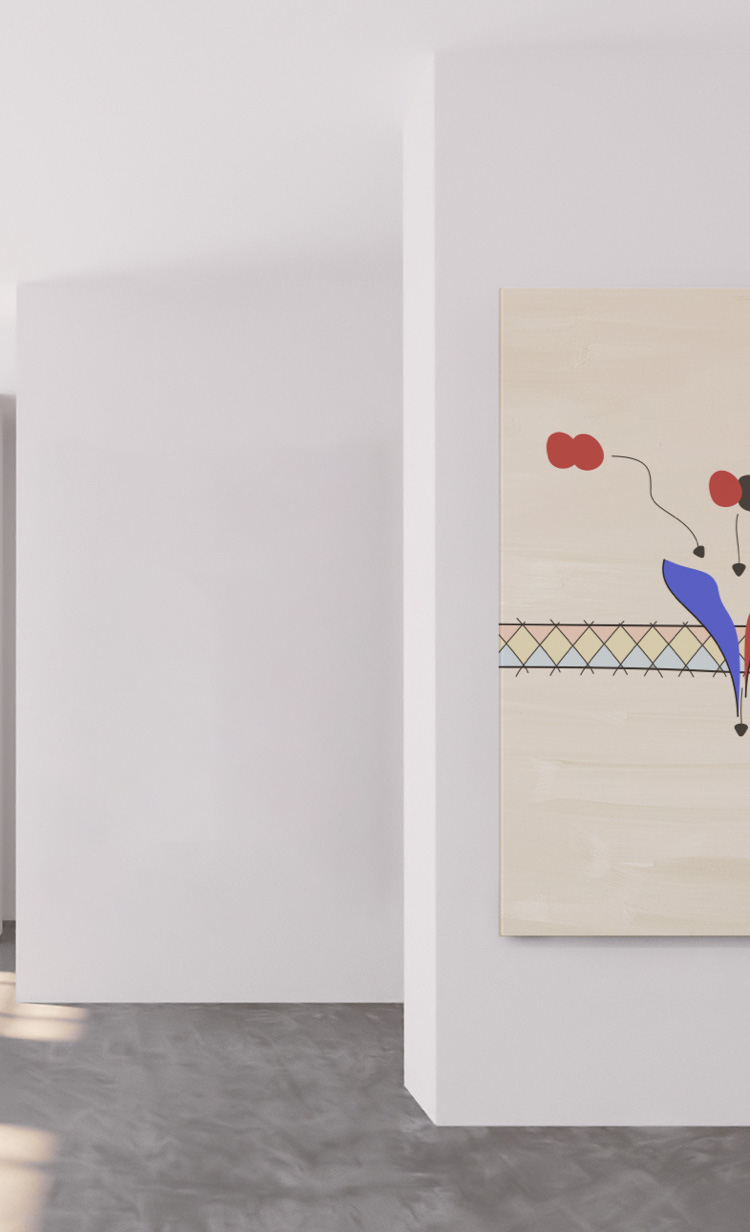
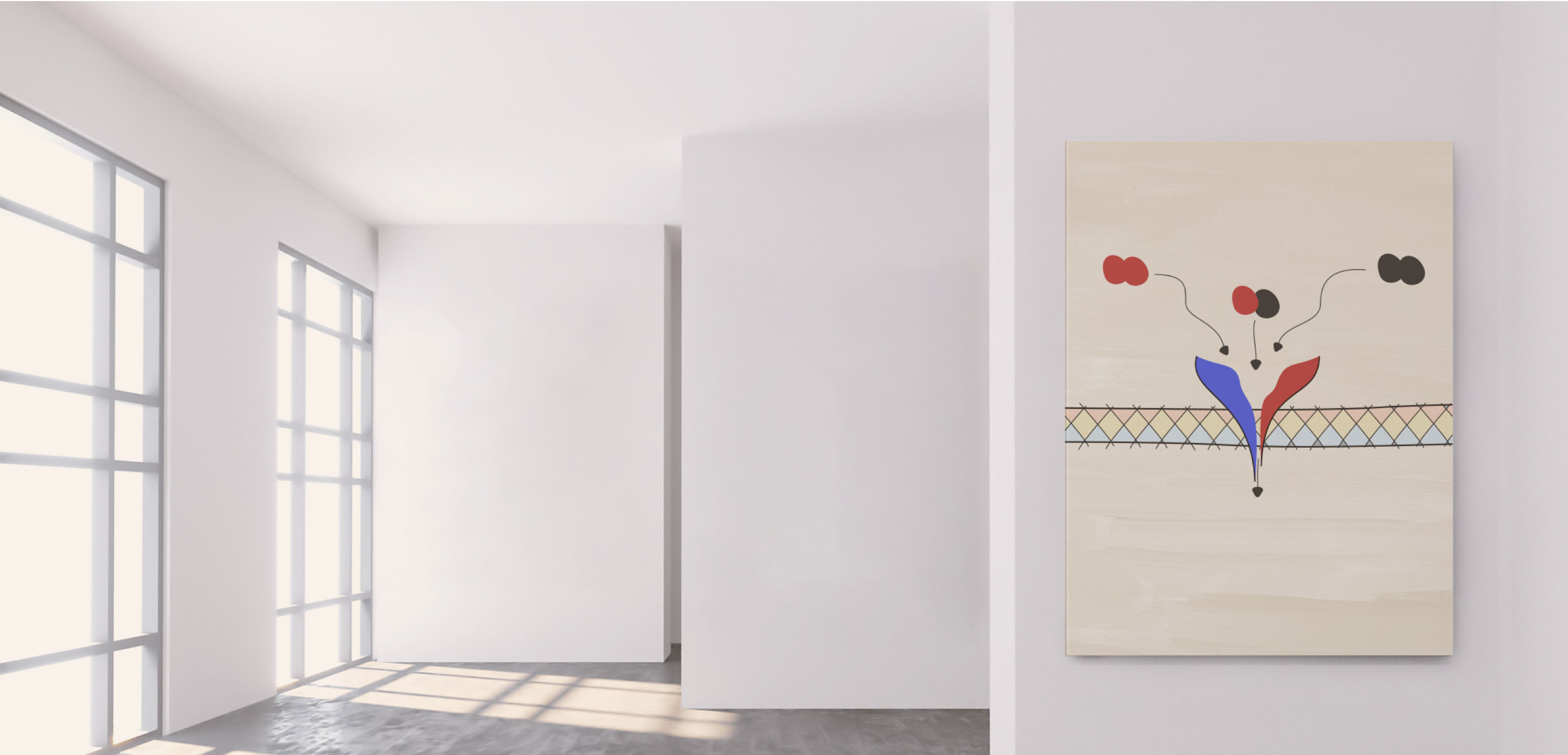
STEP INTO THE FIRST EXHIBIT
Explore the pathobiology of PsA
PsA= psoriatic arthritis.
The chronic inflammatory pathogenesis of PsA is complex and multifactorial…
…involving multiple adaptive and innate immune cells and pro-inflammatory cytokines.1
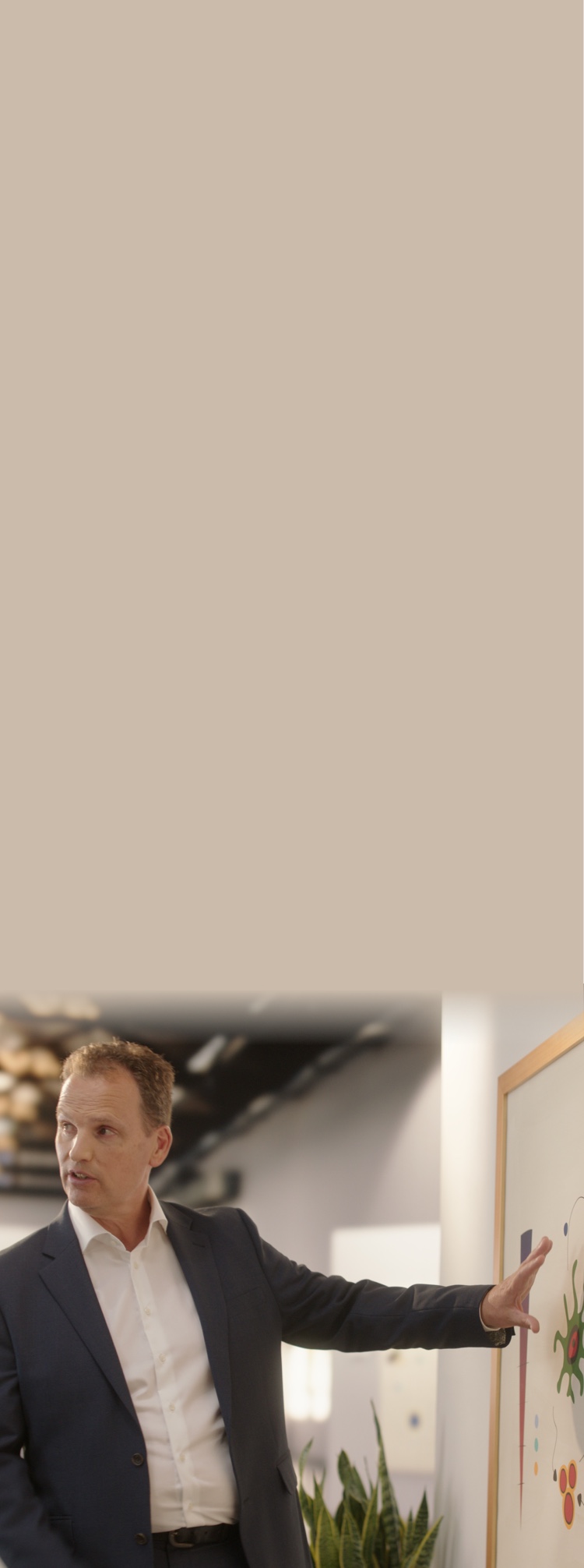
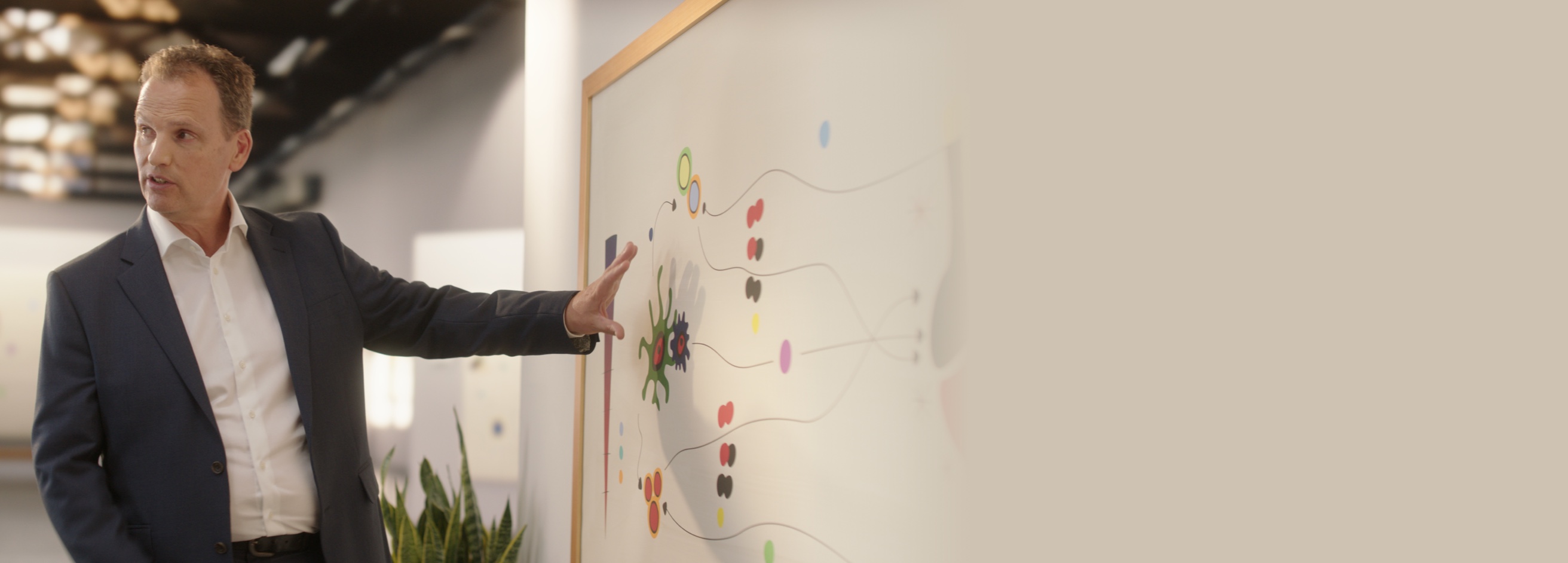
LECTURE SERIES WITH PROF. IAIN MCINNES
Get insights on novel discoveries about cytokines and their role in PsA
Watch a video featuring Prof. Iain McInnes, renowned researcher and clinician in the field of rheumatology, discussing how advancements in molecular medicine have revolutionized our understanding of PsA.
The pathologic processes leading to PsA
The pathologic processes leading to PsA are not yet completely understood. However, recent studies have revealed that there is an intricate interplay of cytokine pathways and cells responsible for initiating and maintaining the chronic inflammation associated with its various clinical manifestations.1-4 Pathology in PsA often differs by clinical manifestation.1,2
The role of cytokines in driving inflammation
The latest evidence shows that several different cytokines, including IL-17A, IL-17F, TNF, IL-12, IL-23 as well as direct or indirect involvement of the JAK/STAT pathway and PDE4, have distinct roles in driving inflammation in different tissues in SpA. Although the relative contributions of each is complex, important distinctions are emerging about which cytokines contribute to the various clinical manifestations and SpA disease phenotypes.4,5,6,7-9
Download a key to help guide you through each piece
JAK=Janus kinase.
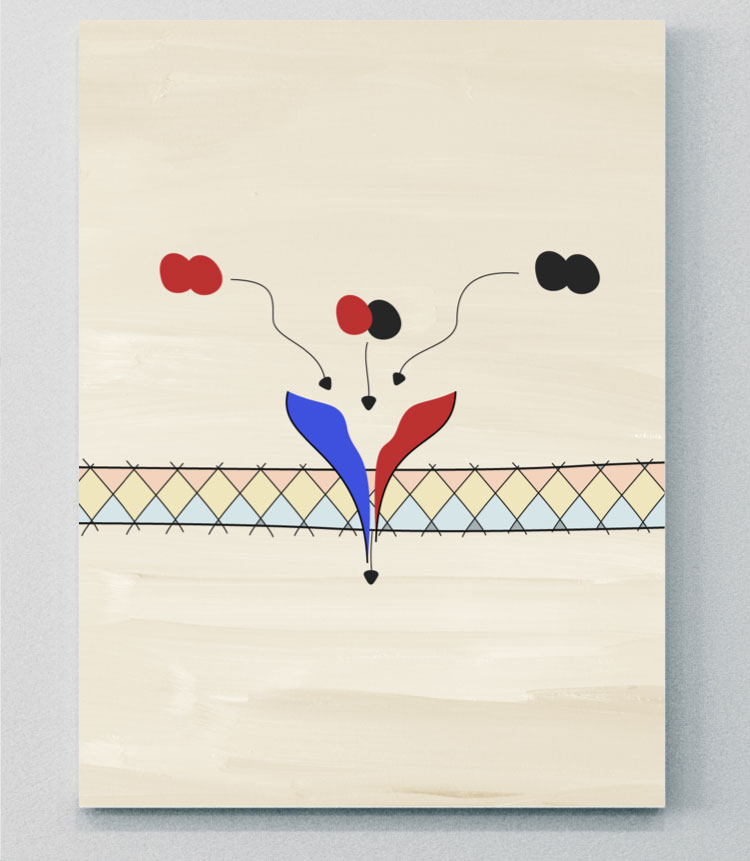
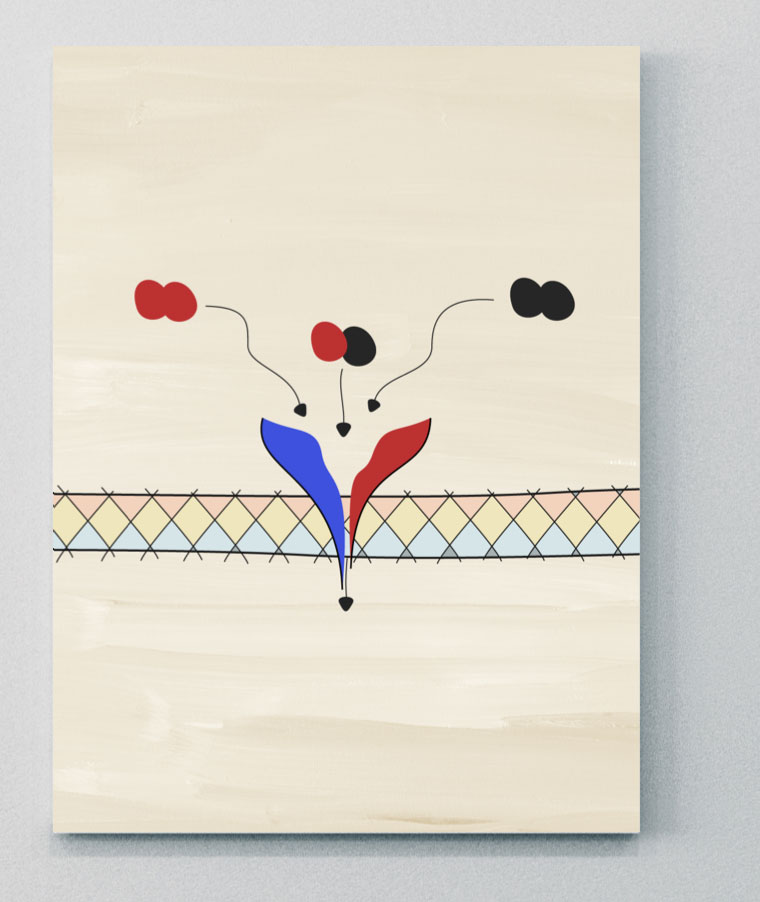
IL-17 family
The IL-17 cytokine family consists of six members that include IL-17A, IL-17B, IL-17C, IL-17D, IL-17E, and IL-17F.10,14
IL-17A and IL-17F share ~50% structural homology and have a similar pro-inflammatory function, signaling via the same receptor complex.13 Studies have shown that IL-17A and IL-17F exist as both homo- and heterodimers, meaning three cytokines exist (IL-17A/A, IL-7A/F, and IL-17F/F), all of which have been shown to contribute to inflammation in PsA.13,15
There are 5 members of the IL-17 receptor family. Two IL-17 receptors (IL-17RC and IL-17RA) are thought to act in concert to mediate signaling by IL-17A/A, IL-17F/F and IL-17A/F.12,14
Studies have shown that IL-17A and IL-17F cooperate with other mediators of inflammation, such as TNF, to amplify inflammatory responses.13 The contribution of IL-17A and IL-17F to pathological bone formation has been shown in a model of osteogenic differentiation, indicating the importance of IL-17A and IL-17F in bone pathogenesis.11
In PsA, skin lesions and inflamed synovia have similar patterns of IL-17A and IL-17F expression and upregulation, and although IL-17A is more potent than IL-17F, the protein level of IL-17F is ~30 times higher than that of IL-17A.16
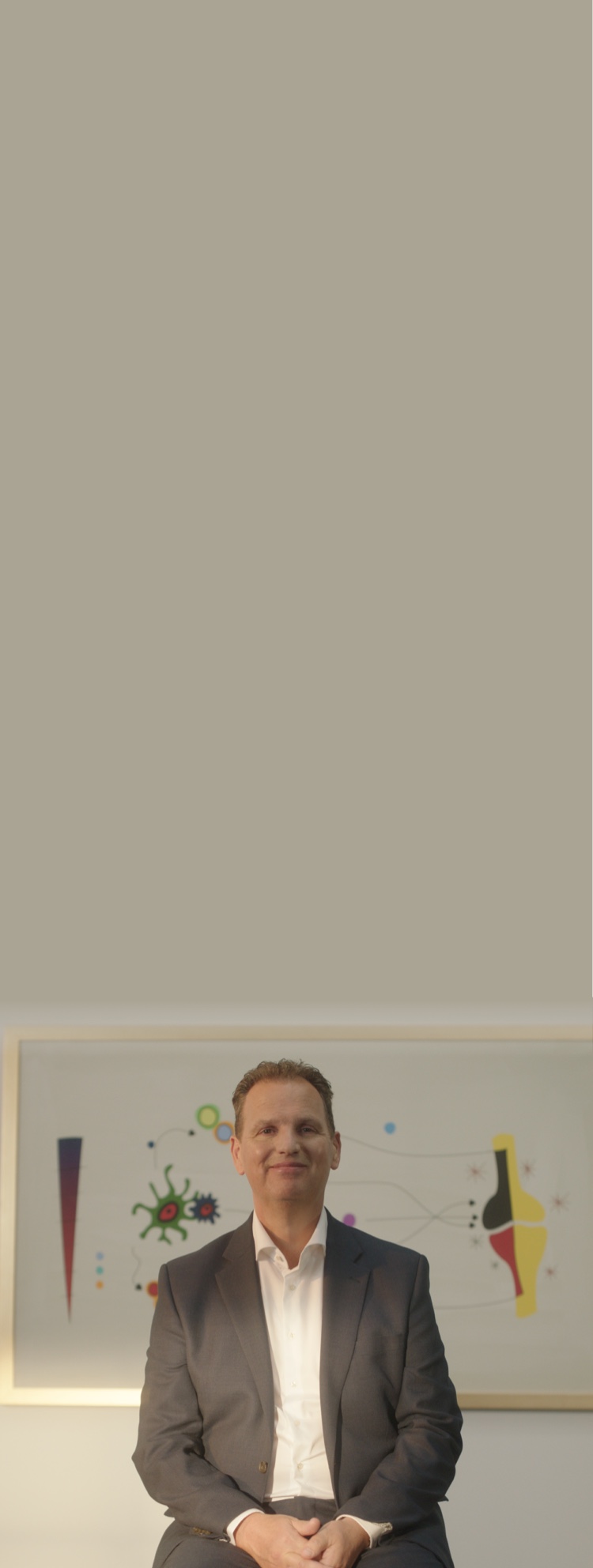
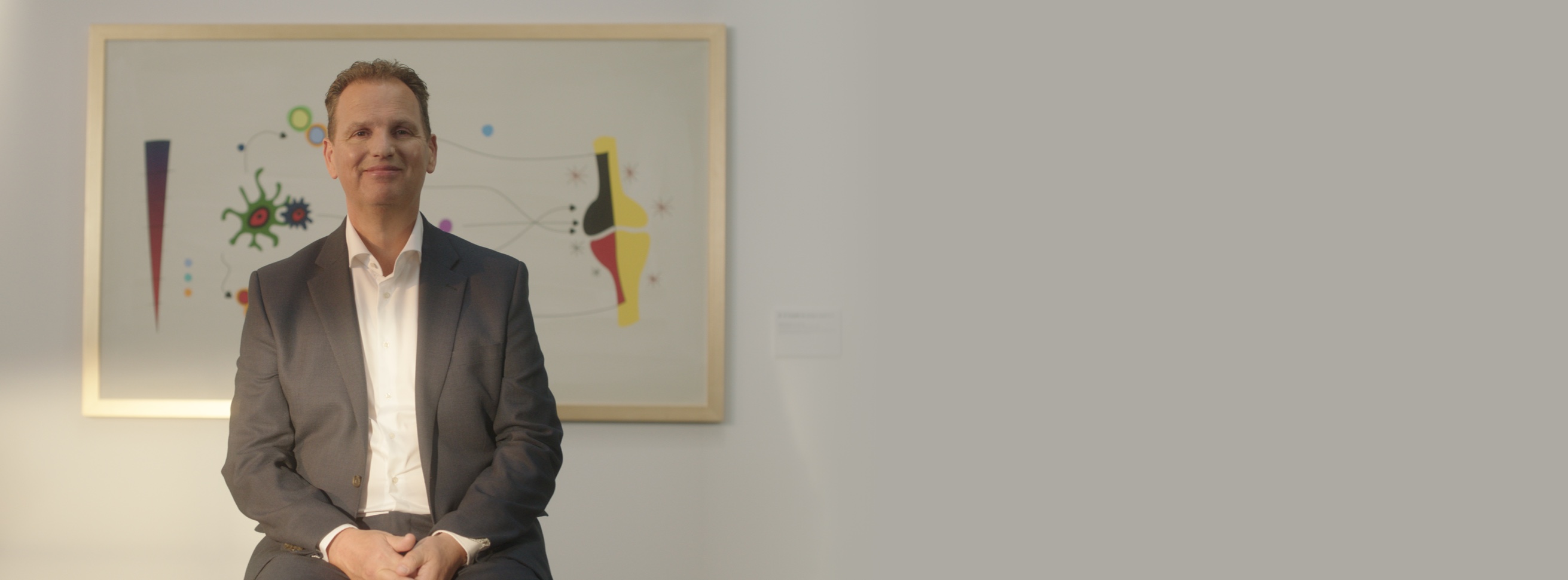
LECTURE SERIES WITH PROF. IAIN MCINNES
Gain a deeper understanding of the role of the IL-23–IL-17 axis in the pathogenesis of PsA
Prof. Iain McInnes delves into the latest science on the varying roles of different cytokines within the IL-23–IL-17 axis in driving inflammation in different tissues.
Evidence suggests IL-17 is regulated by both IL-23-dependent and IL-23-independent pathways
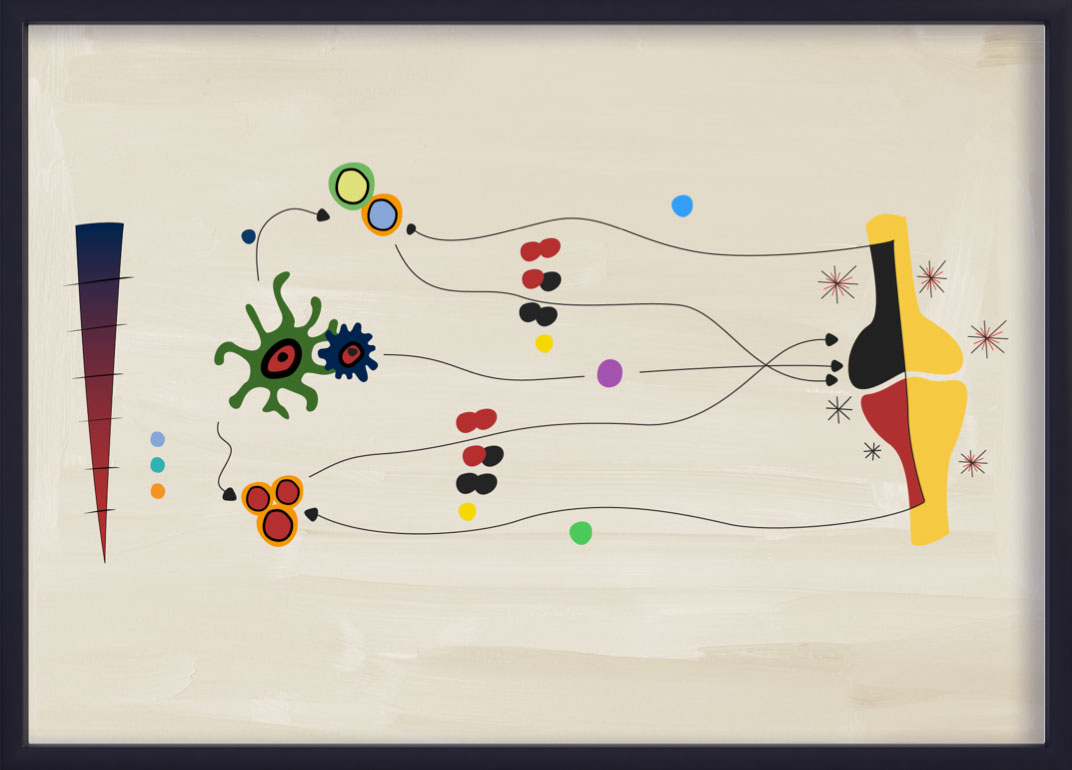
The IL-23–IL-17 axis process
IL-23 is responsible for promoting the expansion and survival of Th17 subsets, including the production of IL-17A and IL-17F from Th17 cells. This process is commonly referred to as the IL-23–IL-17 axis, and has been implicated in several inflammatory diseases, including PsA.14,15,20,21
Recent evidence suggests that the IL-23–IL-17 axis is not a linear “cascade” in PsA.22 IL-23 and IL-17 display partially overlapping but distinct biology and pathobiology.22 Although the IL-23–IL-17 adaptive immunity pathway is still thought to be a key driver of inflammation in PsA, cells of the innate immune system can also produce IL-17A and IL-17F independently of IL-23.2o,22The adaptive cell signaling pathway in PsA is largely driven by IL-23.21,23
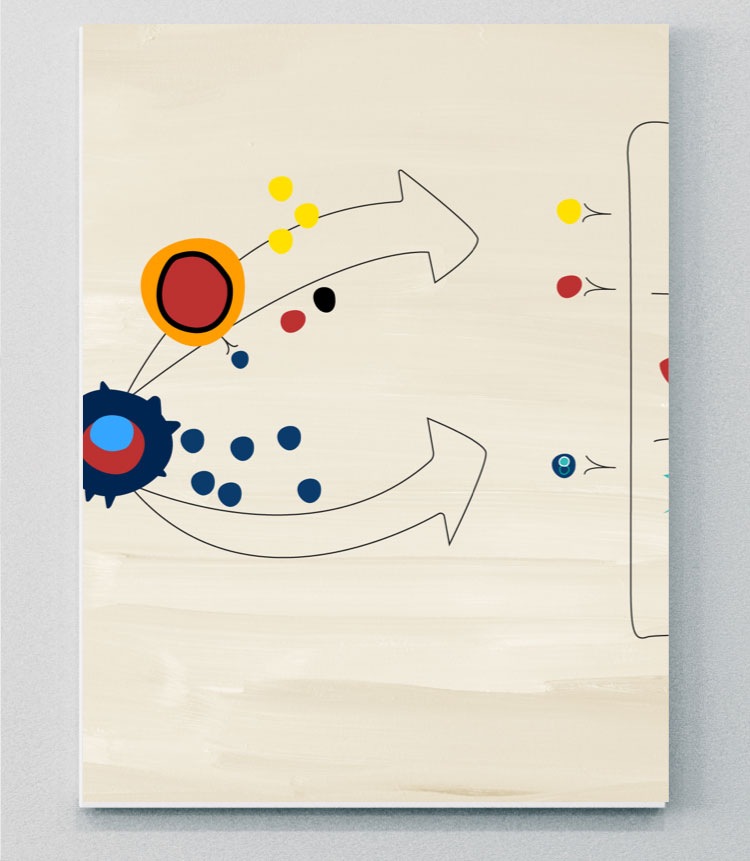
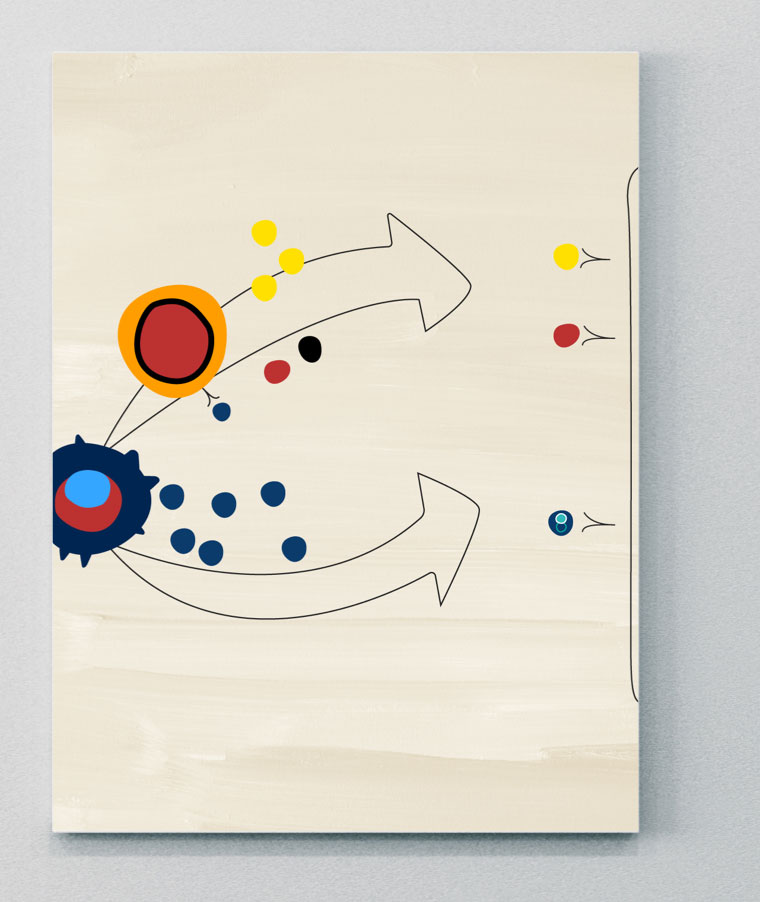
IL-23
IL-23 is an important upstream regulator of IL-17 in PsA14,22
IL-23 is a member of the IL-12 superfamily and is a heterodimer consisting of both a p40 and p19 chain.14,21 It is an important cytokine involved in the pathogenesis of several immune mediated inflammatory diseases, including psoriasis, PsA, axSpA and IBD.21 However, its interaction with different cells and downstream cytokine pathways may be different across diseases.21
There is some evidence to show that IL-23 can migrate from barrier sites such as the gut and skin, where dendritic cells have been activated, and travel to sites of disease pathogenesis in PsA, although this needs to be further explored.21
A key pro-inflammatory role of IL-23 is stimulation of Th17 cells to produce IL-17, IL-22, and TNF, which subsequently leads to downstream inflammation, bone formation and bone erosion.6,14,21 However, recent evidence has shown that IL-23 may also have a direct role in signaling in PsA.21,23 The dependency on IL-23 in driving pathogenic signaling appears to be different across different inflammatory diseases, and direct IL-23 signaling may have a more prominent role in PsA versus axSpA.21,23 This could be related to the different sites of cytokine expression. For example, expression of cytokines linked to the IL-23–IL-17 axis have been shown to be continuously expressed in diseased skin, but there is variability in its expression in the joints.6,21,23
IL-23 acts on numerous target cells via either an IL-17-dependent or an IL-17-independent mechanism, which both lead to the recruitment of inflammatory cells within the inflamed tissue.23
- IL-17-dependent pathway: IL-23 stimulates Th17 cells via IL-23R and induces the release of molecules such as IL-17 or IL-22. By binding their cognate receptors IL-17R or IL-22R, eventually activate the “effector cells” keratinocytes, B cells, osteoclast precursors, macrophages and fibroblast-like synoviocytes.23
- IL-17-independent pathway: The same subset of target cells can be directly challenged by IL-23 in an IL-17-independent manner, via IL-23R.23
IBD=inflammatory bowel disease.
The role of IL-23 in the pathobiology of axSpA is unclear, as clinical trials of biologics blocking this cytokine have failed.
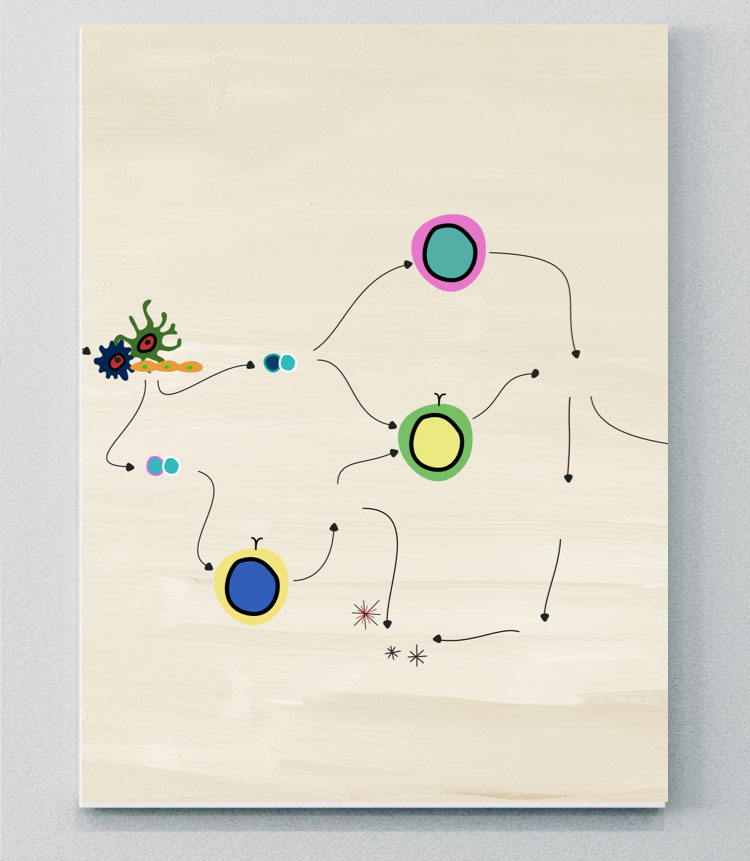
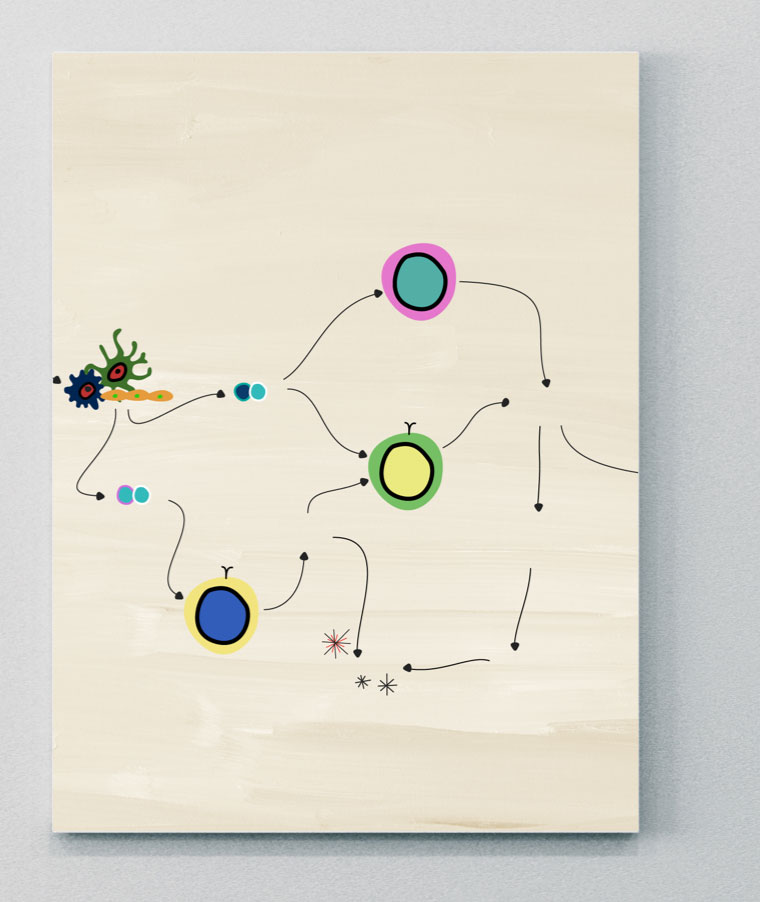
IL-12
Role of IL-12 in PsA
IL-12 is a pro-inflammatory cytokine composed of two subunits (p35 and p40), resulting in an active heterodimer p70.26 Produced by innate immune cells, it induces naïve T cell differentiation into IFN-gamma producing Th1 cells and prevents T cell exhaustion.26,27
IL-12 and IL-23 activity is tightly linked. Both of these inflammatory cytokines share the p40 subunit and a receptor chain (IL-12R1), which controls the distinct IL-12 and IL-23 signaling pathways.26
In PsA, over-activation of IL-12 and IL-23 increases the number of circulating Th1 and Th17 cells. This activates signaling from cytokines and proteins, which can lead to the development of psoriatic plaques.28,29 Susceptibility to PsA has been linked to single nucleotide polymorphisms in the IL-12b gene, which encodes the ligand subunit of IL-23R.26
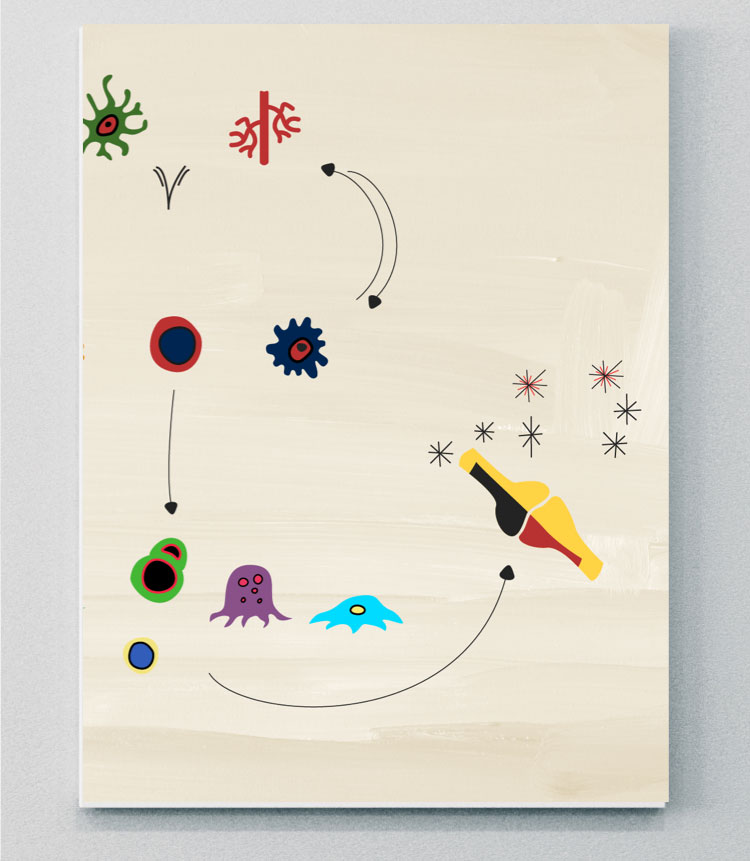
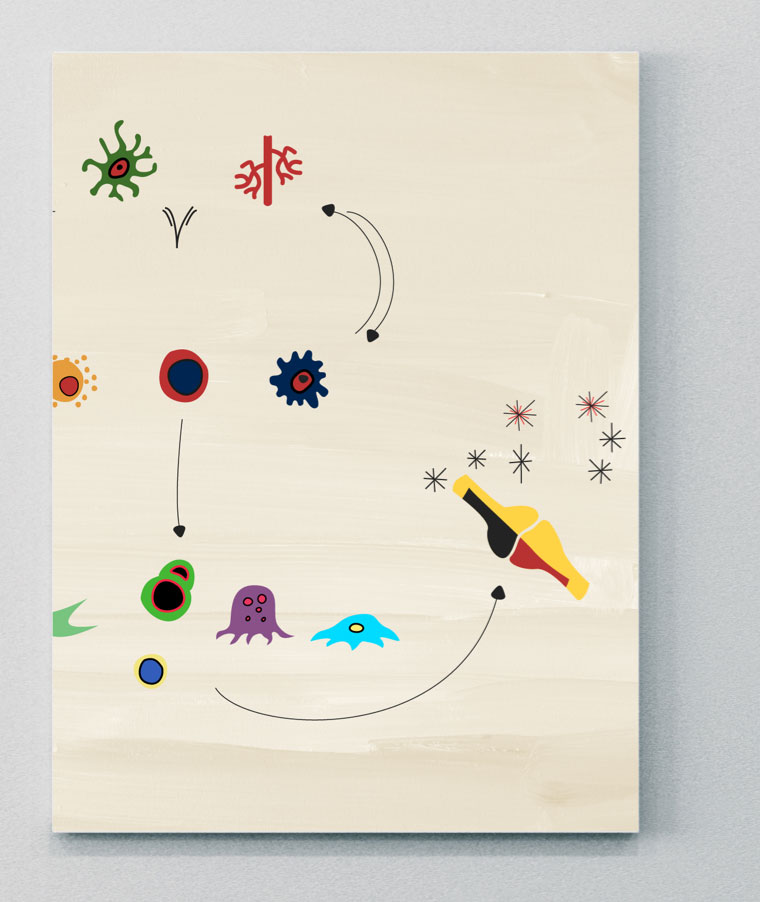
TNF
TNF signaling plays a role in the pathogenic processes of PsA
The TNF superfamily (TNFSF) consists of 19 structurally related pleotropic cytokines.31,32 TNFSF proteins are important drivers of inflammation and play roles in the mediation of apoptosis, angiogenesis, cell proliferation, and other critical biologic functions.31
TNF-α exists in transmembrane and soluble forms and is mostly created by T-cells, NK cells, and macrophages.33 TNF-α cellular signaling occurs through two receptors TNF-receptor1 (TNFR1) and TNFR2, which have differing mechanisms of cellular signaling, ligand affinity, and patterns of expression.34,35 TNFR1 is the dominant receptor involved in inflammatory and innate immune responses.36
The overexpression of TNF drives a pathogenic pro-inflammatory state thought to be mediated by Th1 and Th17 cells.32 Elevated levels of TNF can be found in the synovium and other locations known for skin manifestations, as well as entheses, joint and spine involvement.30,37 Activated dendritic cells secrete TNF-α and other pro-inflammatory cytokines (e.g., IL-6, IL-12, IL-23) which leads to increased T-cell signaling and Th cell differentiation.32 Th1 and Th17 cells upregulate pro-synovial inflammatory cytokine production, which leads to production of osteoclasts, bone/cartilage erosion, and abnormal bone formation.38
In PsA, TNF can synergize with IL-17 and IL-23 pathways:
- IL-23 stimulates Th17 cell differentiation, which leads to the production of cytokines such as IL-17, and causes further upregulation of TNF35
- IL-17 combines with TNF, leading to stronger pro-inflammatory cytokine expression than either cytokine can enact alone39
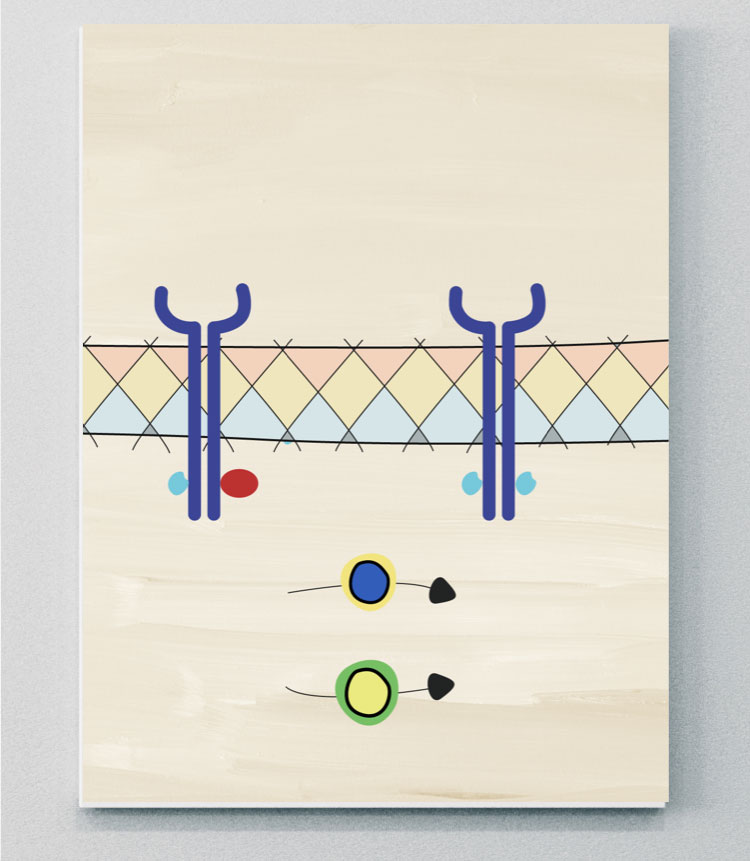
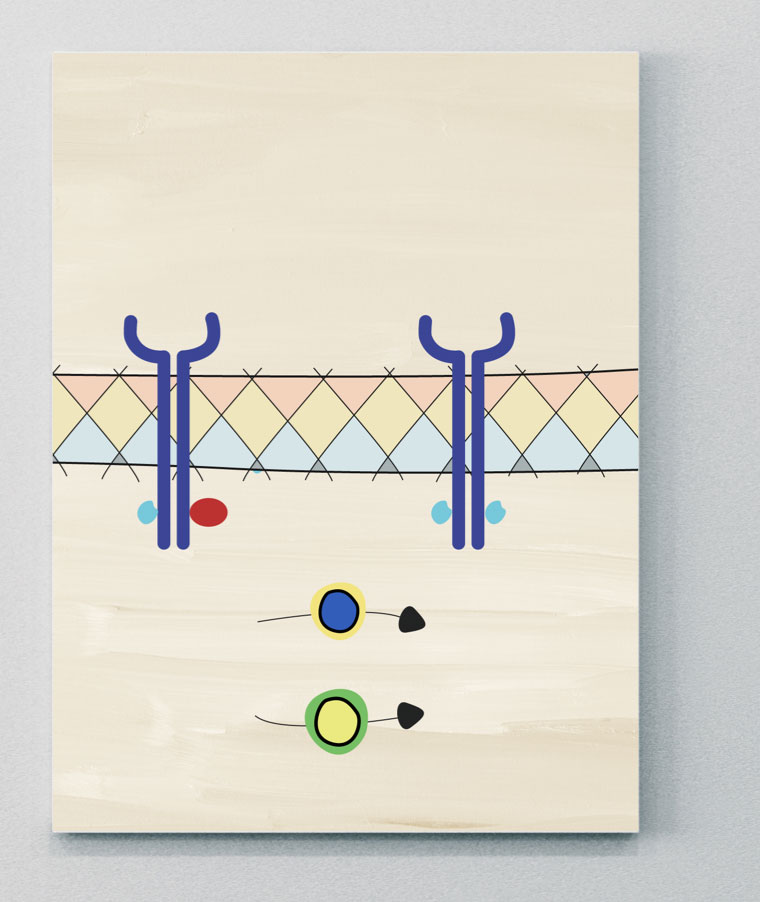
JAK-STAT
JAK-STAT pathways may play an important role in the pathogenesis of PsA
JAK molecules are a group of intracellular tyrosine kinases, comprising four isoforms: JAK1, JAK2, JAK3 and tyrosine kinase (TYK) 2.41 These groups are coupled to signal transducer and activator of transcription (STAT) molecules.9 Many immune cells and effector molecules use different combinations of JAK and STAT molecules to convert signals from the cell surface to the nucleus, where they activate transcription and induce gene activation. This downstream gene activation has a role in regulating different biological processes, including the activation of pathological pathways in PsA.9,40,42 Many SpA-associated cytokines are mediated either directly or indirectly via JAK-STAT pathways.40
JAK=Janus kinase; TYK=tyrosine kinase.
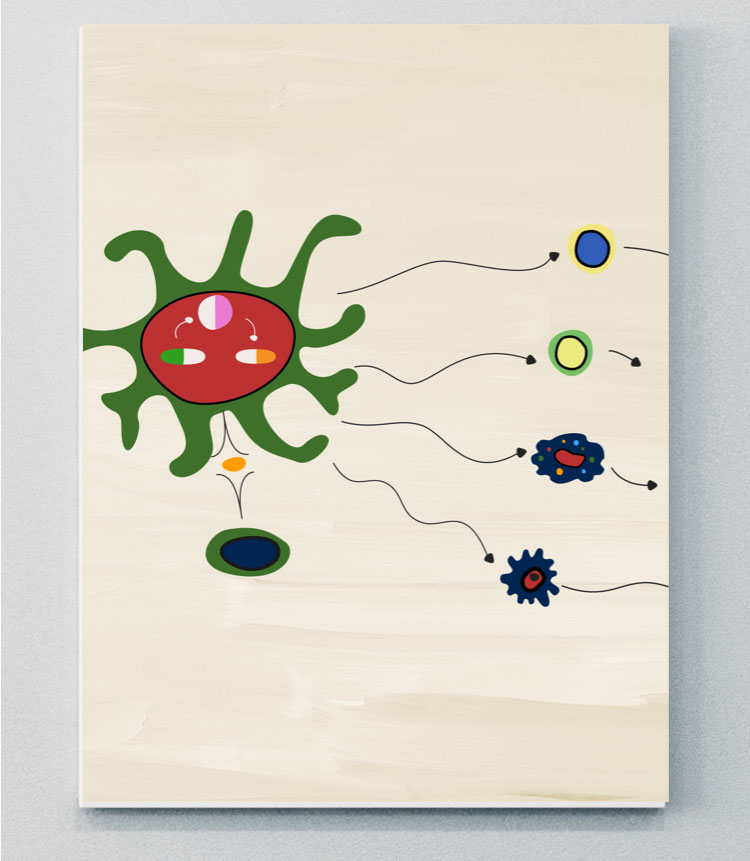
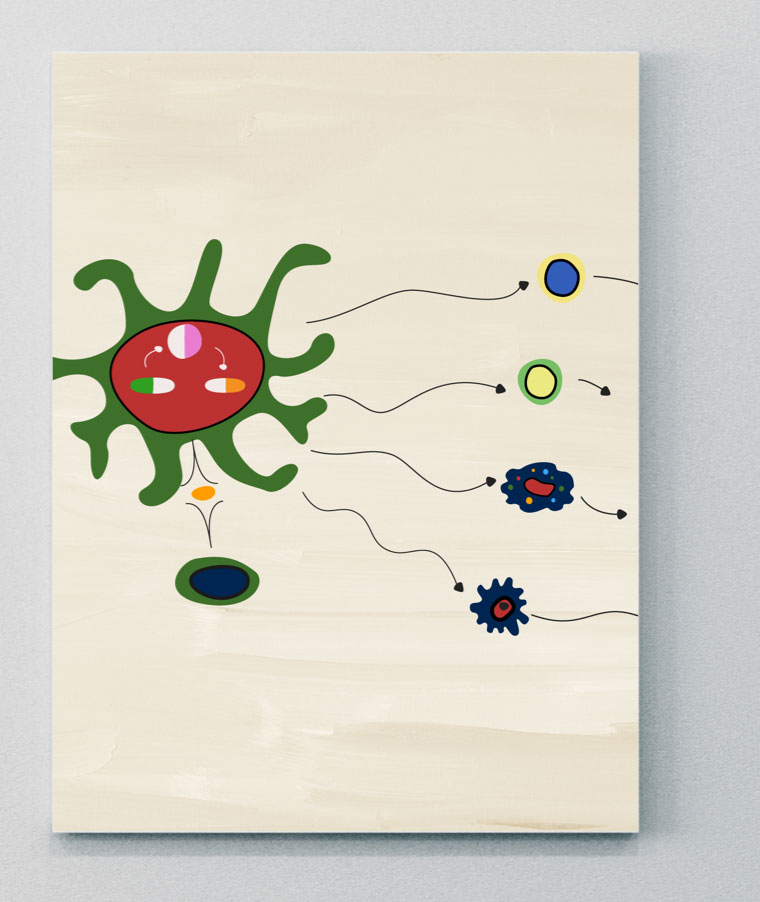
PDE4
A cellular mediator of the inflammatory response leading to PsA
PDE4 is a member of the phosphodiesterase enzyme family, which catalyze the degradation of intracellular second messengers, including cAMP and cGMP, and are involved in a diverse range of inflammatory mechanisms.4
In PsA, cAMP regulation is impaired, which is thought to be driven by increases in PDE4, which catalyze the hydrolysis of cAMP to AMP.4,43 Increases in AMP levels can, in turn, lead to an inflammatory cytokine imbalance (i.e., increases in inflammatory cytokine expression of TNF, IL-17, IFN, IL-23, and reductions in regulatory cytokines, such as IL-10), which drive inflammatory pathways leading to pathogenic bone formation and erosion.4,44
PDE=phosphodiesterase.
Take your seats in the Animated Science Theatre
Uncover the mechanisms driving PsA, including the role of IL-17A and IL-17F
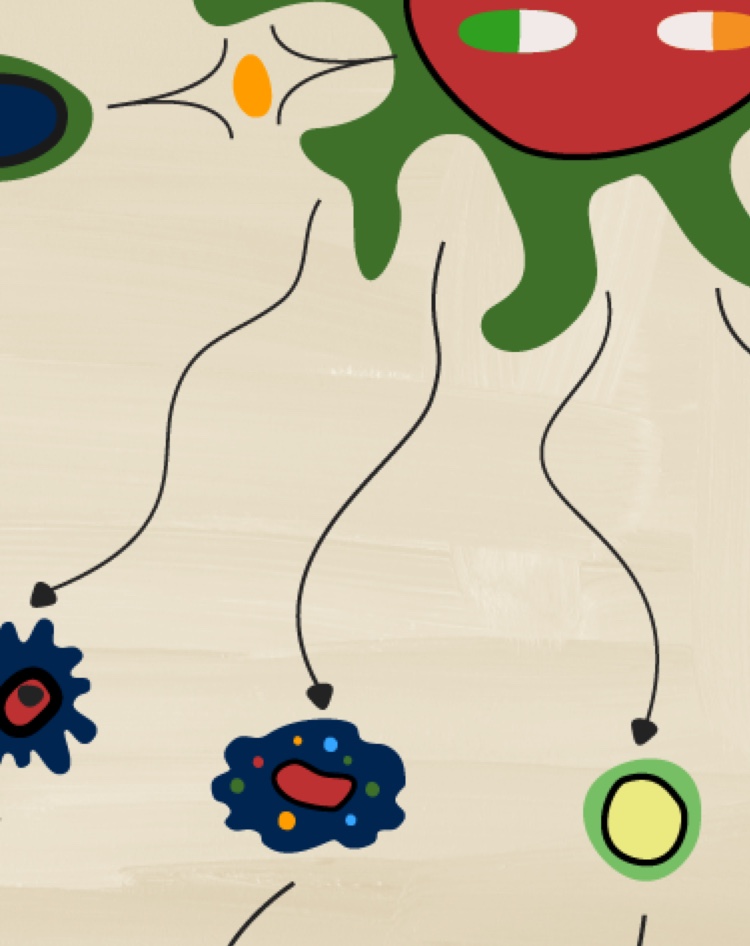

Discover how several different cytokines have distinct roles in driving inflammation in axSpA
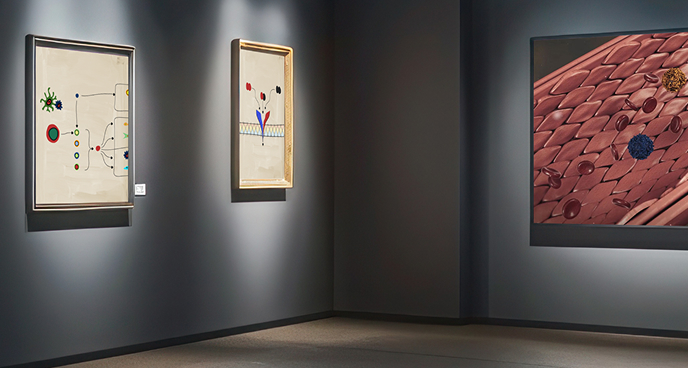
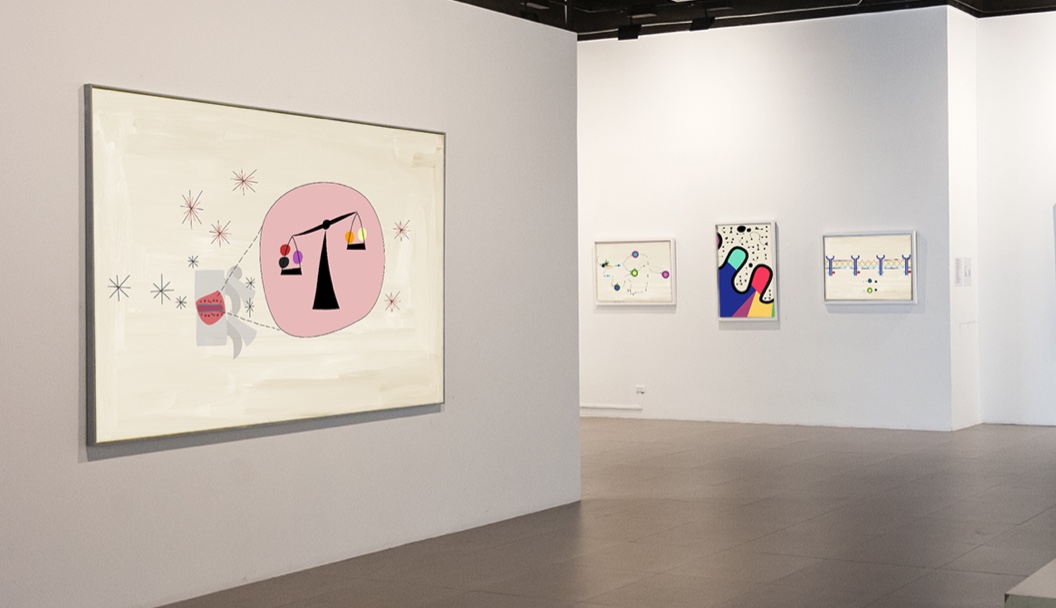
NEXT ROOM IN THE PSA EXHIBIT
Immune dysregulation
Explore how cytokine dysregulation drives inflammation in PsA.
Become a member of the RheuMuseum and be the first to know about new exhibits
- Veale DJ, Fearon U. The pathogenesis of psoriatic arthritis. Lancet. 2018;391(10136):2273-2284. doi:10.1016/S0140-6736(18)30830-4
- Bravo A, Kavanaugh A. Bedside to bench: defining the immunopathogenesis of psoriatic arthritis. Nat Rev Rheumatol. 2019;15(11):645-656. doi:10.1038/s41584-019-0285-8
- Crispino N, Ciccia F. JAK/STAT pathway and nociceptive cytokine signaling in rheumatoid arthritis and psoriatic arthritis. Clin Exp Rheumatol. 2021;39(3):668-675
- Picchianti-Diamanti A, Spinelli FR, Rosado MM, et al. Inhibition of phosphodiesterase-4 in psoriatic arthritis and inflammatory bowel diseases. Int J Mol Sci. 2021;22(5):2638. Published 2021 Mar 5. doi:10.3390/ijms22052638
- Rezaiemanesh A, Abdolmaleki M, Abdolmohammadi K, et al. Immune cells involved in the pathogenesis of ankylosing spondylitis. Biomed Pharmacother. 2018;100:198-204. doi:10.1016/j.biopha.2018.01.108
- McGonagle DG, McInnes IB, Kirkham BW, et al. The role of IL-17A in axial spondyloarthritis and psoriatic arthritis: recent advances and controversies [published correction appears in Ann Rheum Dis. 2020;79(1):e12]. Ann Rheum Dis. 2019;78(9):1167-1178. doi:10.1136/annrheumdis-2019-215356
- Siebert S, Millar NL, McInnes IB. Why did IL-23p19 inhibition fail in AS: a tale of tissues, trials or translation?. Ann Rheum Dis. 2019;78(8):1015-1018. doi:10.1136/annrheumdis-2018-213654
- Rosine N, Miceli-Richard C. Innate cells: the alternative source of IL-17 in axial and peripheral spondyloarthritis?. Front Immunol. 2021;11:553742. Published 2021. doi:10.3389/fimmu.2020.553742
- Hammitzsch A, Lorenz G, Moog P. Impact of janus kinase inhibition on the treatment of axial spondyloarthropathies. Front Immunol. 2020;11:591176. Published 2020. doi:10.3389/fimmu.2020.591176
- Yang XO, Chang SH, Park H, et al. Regulation of inflammatory responses by IL-17F. J Exp Med. 2008;205(5):1063-1075. doi:10.1084/jem.20071978
- Shah M, Maroof A, Gikas P, et al. Dual neutralisation of IL-17F and IL-17A with bimekizumab blocks inflammation-driven osteogenic differentiation of human periosteal cells. RMD Open. 2020;6(2):e001306. doi:10.1136/rmdopen-2020-001306
- Goepfert A, Lehmann S, Blank J, et al. Structural Analysis Reveals that the Cytokine IL-17F Forms a Homodimeric Complex with Receptor IL-17RC to Drive IL-17RA-Independent Signaling. Immunity. 2020;52(3):499-512.e5. doi:10.1016/j.immuni.2020.02.004
- Glatt S, Baeten D, Baker T, et al. Dual IL-17A and IL-17F neutralisation by bimekizumab in psoriatic arthritis: evidence from preclinical experiments and a randomised placebo-controlled clinical trial that IL-17F contributes to human chronic tissue inflammation. Ann Rheum Dis. 2018;77(4):523-532. doi:10.1136/annrheumdis-2017-212127
- Tsukazaki H, Kaito T. The role of the IL-23/IL-17 pathway in the pathogenesis of spondyloarthritis. Int J Mol Sci. 2020;21(17):6401. Published 2020. doi:10.3390/ijms21176401
- Bridgewood C, Sharif K, Sherlock J, et al. Interleukin-23 pathway at the enthesis: The emerging story of enthesitis in spondyloarthropathy. Immunol Rev. 2020;294(1):27-47. doi:10.1111/imr.12840
- Ritchlin CT, Kavanaugh A, Merola JF, et al. Bimekizumab in patients with active psoriatic arthritis: results from a 48-week, randomised, double-blind, placebo-controlled, dose-ranging phase 2b trial. Lancet. 2020;395(10222):427-440. doi:10.1016/S0140-6736(19)33161-7
- Blanco P, Palucka AK, Pascual V, et al. Dendritic cells and cytokines in human inflammatory and autoimmune diseases. Cytokine Growth Factor Rev. 2008;19(1):41-52. doi:10.1016/j.cytogfr.2007.10.004
- Cole S, Murray J, Simpson C, et al. Interleukin (IL)-12 and IL-18 synergize to promote MAIT cell IL-17A and IL-17F production independently of IL-23 signaling. Front Immunol. 2020;11:585134. Published 2020. doi:10.3389/fimmu.2020.585134
- Russell T, Watad A, Bridgewood C, et al. IL-17A and TNF modulate normal human spinal entheseal bone and soft tissue mesenchymal stem cell osteogenesis, adipogenesis, and stromal function. Cells. 2021;10(2):341. Published 2021. doi:10.3390/cells10020341
- McGonagle D, Watad A, Sharif K, et al. Why inhibition of IL-23 lacked efficacy in ankylosing spondylitis. Front Immunol. 2021;12:614255. Published 2021. doi:10.3389/fimmu.2021.614255
- Mease P, Van den Bosch F. IL-23 and axial disease: do they come together? Rheumatology. 2021;60(Suppl 4):iv28-iv33. doi:10.1093/rheumatology/keab617
- Baeten D, Adamopoulos IE. IL-23 inhibition in ankylosing spondylitis: where did it go wrong?. Front Immunol. 2021;11:623874. Published 2021. doi:10.3389/fimmu.2020.623874
- Boutet MA, Nerviani A, Gallo Afflitto G, et al. Role of the IL-23/IL-17 axis in psoriasis and psoriatic arthritis: the clinical importance of its divergence in skin and joints. Int J Mol Sci. 2018;19(2):530. Published 2018. doi:10.3390/ijms19020530
- Taams LS, Steel KJA, Srenathan U, et al. IL-17 in the immunopathogenesis of spondyloarthritis. Nat Rev Rheum. 2018;14(8):453–466. Published 2018. doi: 10.1038/s41584-018-0044-2
- Zhu W, He X, Cheng K, et al. Ankylosing spondylitis: etiology, pathogenesis, and treatments. Bone Res. 2019;7:22. Published 2019. doi:10.1038/s41413-019-0057-8
- Schurich A, Raine C, Morris V, et al. The role of IL-12/23 in T cell-related chronic inflammation: implications of immunodeficiency and therapeutic blockade. Rheumatology (Oxford). 2018;57(2):246-254. doi:10.1093/rheumatology/kex186
- Trinchieri G. Interleukin-12: a proinflammatory cytokine with immunoregulatory functions that bridge innate resistance and antigen-specific adaptive immunity. Annu Rev Immunol. 1995;13:251-276. doi:10.1146/annurev.iy.13.040195.001343
- Johnsson HJ, McInnes IB. Interleukin-12 and interleukin-23 inhibition in psoriatic arthritis. Clin Exp Rheumatol. 2015;33(5 Suppl 93):S115-S118
- Chyuan IT, Lai JH. New insights into the IL-12 and IL-23: From a molecular basis to clinical application in immune-mediated inflammation and cancers. Biochem Pharmacol. 2020;175:113928. doi:10.1016/j.bcp.2020.113928
- Silvagni E, Missiroli S, Perrone M, et al. From bed to bench and back: TNF-α, IL-23/IL-17A, and JAK-dependent inflammation in the pathogenesis of psoriatic synovitis. Front Pharmacol. 2021;12:672515. Published 2021. doi:10.3389/fphar.2021.672515
- Aggarwal BB, Gupta SC, Kim JH. Historical perspectives on tumor necrosis factor and its superfamily: 25 years later, a golden journey. Blood. 2012;119(3):651-665. doi:10.1182/blood-2011-04-325225
- Croft M, Siegel RM. Beyond TNF: TNF superfamily cytokines as targets for the treatment of rheumatic diseases. Nat Rev Rheumatol. 2017;13(4):217-233. doi:10.1038/nrrheum.2017.22
- Jang DI, Lee AH, Shin HY, et al. The role of tumor necrosis factor alpha (TNF-α) in autoimmune disease and current TNF-α inhibitors in therapeutics. Int J Mol Sci. 2021;22(5):2719. Published 2021. doi:10.3390/ijms22052719
- Holbrook J, Lara-Reyna S, Jarosz-Griffiths H, McDermott M. Tumour necrosis factor signaling in health and disease. F1000Res. 2019;8:F1000 Faculty Rev-111. Published 2019. doi:10.12688/f1000research.17023.1
- Mantravadi S, Ogdie A, Kraft WK. Tumor necrosis factor inhibitors in psoriatic arthritis. Expert Rev Clin Pharmacol. 2017;10(8):899-910. doi:10.1080/17512433.2017.1329009
- Lata M, Hettinghouse AS, Liu CJ. Targeting tumor necrosis factor receptors in ankylosing spondylitis. Ann N Y Acad Sci. 2019;1442(1):5-16. doi:10.1111/nyas.13933
- Addimanda O, Possemato N, Caruso A, et al. The role of tumor necrosis factor-α blockers in psoriatic disease. Therapeutic Options in Psoriatic Arthritis. J Rheumatol Suppl. 2015;93:73-78. doi:10.3899/jrheum.150642
- Liu J, Dong Z, Zhu Q, et al. TNF-α promoter polymorphisms predict the response to etanercept more powerfully than that to infliximab/adalimumab in spondyloarthritis. Sci Rep. 2016;6:32202. Published 2016. doi:10.1038/srep32202
- Noack M, Beringer A, Miossec P. Additive or synergistic interactions between IL-17A or IL-17F and TNF or IL-1β depend on the cell type. Front Immunol. 2019;10:1726. Published 2019. doi:10.3389/fimmu.2019.01726
- McInnes IB, Szekanecz Z, McGonagle D, et al. A review of JAK-STAT signaling in the pathogenesis of spondyloarthritis and the role of JAK inhibition [published online ahead of print, 2021]. Rheumatology (Oxford). 2021;keab740. doi:10.1093/rheumatology/keab740
- White JPE, Coates LC. JAK1 selective inhibitors for the treatment of spondyloarthropathies. Rheumatology (Oxford). 2021;60(Suppl 2):ii39-ii44. doi:10.1093/rheumatology/keaa815
- Fragoulis GE, McInnes IB, Siebert S. JAK-inhibitors. New players in the field of immune-mediated diseases, beyond rheumatoid arthritis. Rheumatology (Oxford). 2019;58(Suppl 1):i43-i54. Published 2019. doi: 10.1093/rheumatology/key276.
- Kilanowska A, Ziółkowska A. Role of phosphodiesterase in the biology and pathology of diabetes. Int J Mol Sci. 2020;21(21):8244. Published 2020. doi:10.3390/ijms21218244
- Li H, Zuo J, Tang W. Phosphodiesterase-4 inhibitors for the treatment of inflammatory diseases. Front Pharmacol. 2018;9:1048. Published 2018. doi:10.3389/fphar.2018.01048